Our monthly PATHS-UP publication list in April 2022. Please read the abstracts below :
The awareness of the individuals’ biological status is critical for creating interactive environments. Accordingly, we devised a multimodal cryptographic bio-human–machine interface (CB-HMI), which seamlessly translates touch-based entries into encrypted biochemical, biophysical, and biometric indices (i.e., circulating biomarkers levels, heart rate, oxygen saturation level, and fingerprint pattern). As its central component, the CB-HMI features thin hydrogel-coated chemical sensors and a signal interpretation framework to access/interpret biochemical indices, bypassing the challenge of circulating analyte accessibility and the confounding effect of pressing force variability. Upgrading the surrounding objects with CB-HMI, we demonstrated new interactive solutions for driving safety and medication use, where the integrated CB-HMI uniquely enabled one-touch bioauthentication (based on the user’s biological state/identity), prior to rendering the intended services.
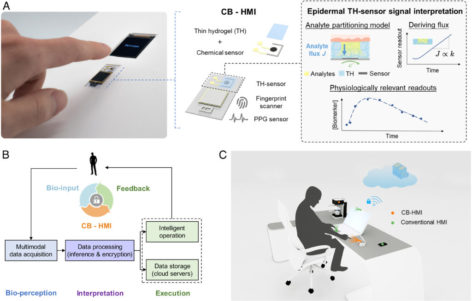
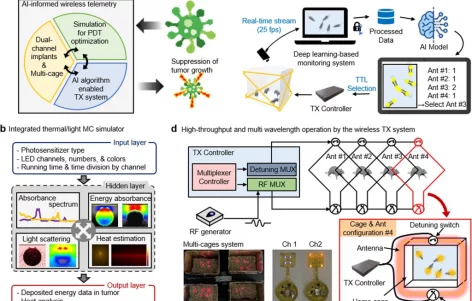
Photodynamic therapy (PDT) offers several advantages for treating cancers, but its efficacy is highly dependent on light delivery to activate a photosensitizer. Advances in wireless technologies enable remote delivery of light to tumors, but suffer from key limitations, including low levels of tissue penetration and photosensitizer activation. Here, we introduce DeepLabCut (DLC)-informed low-power wireless telemetry with an integrated thermal/light simulation platform that overcomes the above constraints. The simulator produces an optimized combination of wavelengths and light sources, and DLC-assisted wireless telemetry uses the parameters from the simulator to enable adequate illumination of tumors through high-throughput (<20 mice) and multi-wavelength operation. Together, they establish a range of guidelines for effective PDT regimen design. In vivo Hypericin and Foscan mediated PDT, using cancer xenograft models, demonstrates substantial suppression of tumor growth, warranting further investigation in research and/or clinical settings.
Malaria is often most endemic in remote regions where diagnostic microscopy services are unavailable. In such regions, the use of rapid diagnostic tests fails to quantify parasitemia measurements which reflect the concentration of Plasmodium parasites in the bloodstream. Thus, novel diagnostic and monitoring technologies capable of providing such information could improve the quality of treatment, monitoring, and eradication efforts. A low-cost, portable microscope for gathering quantitative parasitemia data from fluorescently stained thin blood smears is presented. The system employs bimodal imaging using components optimized for cost savings, system robustness, and optical performance. The microscope is novel for its use of monochromatic visible illumination paired with a long working distance singlet aspheric objective lens that can image both traditionally mounted and cartridge-based blood smears. Eight dilutions of red blood cells containing laboratory cultured wild-type P. falciparum were used to create thin smears which were stained with SYBR Green-1 fluorescent dye. Two subsequent images are captured for each field-of-view, with brightfield images providing cell counts and fluorescence images providing parasite localization data. Results indicate the successful resolution of sub-micron sized parasites, and parasitemia measurements from the prototype microscope display linear correlation with measurements from a benchtop microscope with a limit of detection of 0.18 parasites per 100 red blood cells.
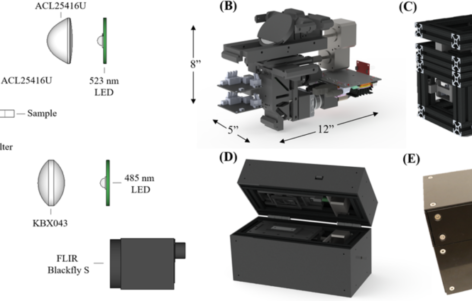